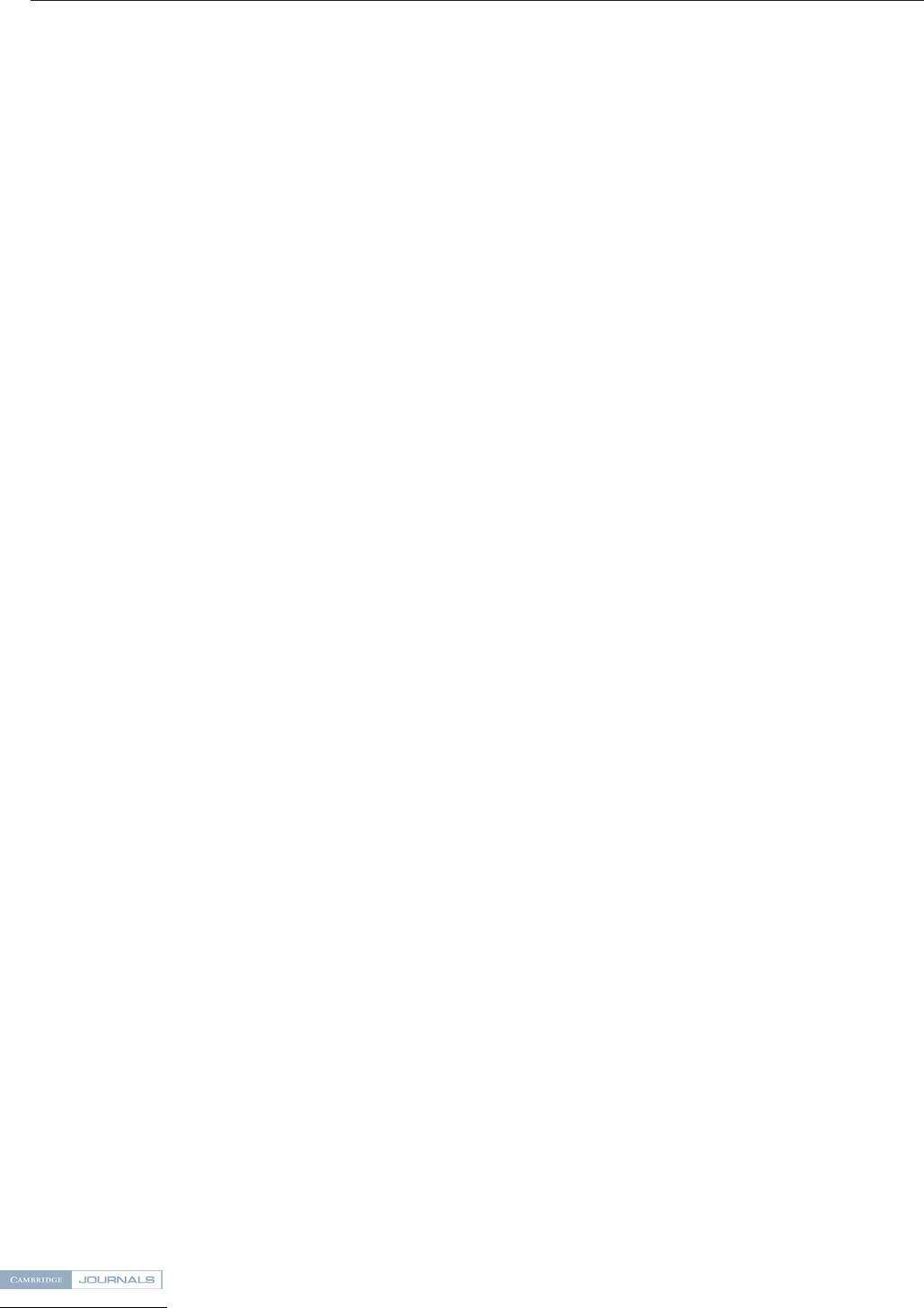
http://journals.cambridge.org Downloaded: 10 Jan 2016 IP address: 132.239.1.230
that the pyridinelike structure is independent of the pre-
cursors used in our experiments. Also, this donor state is
in a fashion similar to that reported by Choi
30
and Nevi-
domskyy et al.
31
A small number of the tubes examined
show spectra as in which the valence band and conduc-
tion band features appear symmetric about the Fermi
level without the additional feature observed exactly like
the spectra from pure carbon nanotubes, as shown in
Fig. 5(c). This suggests that carbon nanotube growth
within the same growth zones may not be completely
heterogeneous. It should be noted that the raising of the
Fermi level due to the graphite like structure
29
is not
observed in our studies, maybe due to lower nitrogen
doping concentration. We do note that the predicted ap-
proximately 0.5 eV feature
30
in the conduction band is
observed in our spectra as a subtle splitting of the van
Hove singular point. However, further study is needed to
make a definite assignment of this feature with graphite-
like substitution N.
IV. CONCLUSIONS
The yield and quality of CN
x
nanotubes grown using
CVD techniques and pyridine or pyimidene, is com-
pletely comparable to that of pure carbon nanotubes by
similar methods and is evidence that such materials could
be produced in large quantities. The observed nitrogen
concentration can be increased through the substitution
of pyrimidine (3.2%) for pyridine (1–2%). However, we
do note that this may not correlate with the total nitrogen
concentration of the precursor since the kinetics and ther-
modynamics of the two precursors are expected to be
entirely different. In both cases the CN
x
nanotubes have
a uniform diameter and bamboo structure when grown at
700 °C and above. From XPS, the nitrogen is thought to
be incorporated into the nanotube lattice in two ways:
pyridinelike and graphitelike structure with the graphite-
like structures preferred at high growth temperatures
(fewer defects). STS further confirms the existence of the
pyridine-like structure. This work indicates that property
modification and engineered electronic structure in car-
bon nanotubes is possible through substitutional doping
schemes using CVD methods.
REFERENCES
1. S. Iijima: Helical microtubules of graphitic carbon. Nature 354, 56
(1991).
2. D.M. Teter and R.J. Hemley: Low-compressibility carbon ni-
trides. Science 271, 53 (1996).
3. Y. Miyamoto, M.L. Cohen, and S.G. Louie: Theoretical investi-
gation of graphitic carbon nitride and possible tubule forms. Solid
State Commun. 102, 605 (1997).
4. E. Hernandez, C. Goze, P. Bernier, and A. Rubio: Elastic prop-
erties of single-wall nanotubes. Appl. Phys. A: Mater. Sci. Proc.
68, 287 (1999).
5. M. Doytcheva, M. Kaiser, M.A. Verheijen, M. Reyes-Reyes,
M. Terrones, and N. de Jonge: Electron emission from individual
nitrogen-doped multi-walled carbon nanotubes. Chem. Phys. Lett.
396, 126 (2004).
6. K. Suenaga, M.P. Johansson, N. Hellgren, E. Broitman,
L.R. Wallenberg, C. Colliex, J-E. Sundgren, and L. Hultman:
Carbon nitride nanotubulite-densely-packed and well aligned tu-
bular nanostructures. Chem. Phys. Lett. 300, 695 (1999).
7. M. Yudasaka, R. Kikuchi, Y. Ohki, and S. Yoshimura: Nitrogen-
containing carbon nanotube growth from Ni phthalocyanine by
chemical vapor deposition. Carbon 35, 195 (1997).
8. S.L. Sung, S.H. Tsai, C.H. Tseng, F.K. Chiang, X.W. Liu, and
H.C. Shih: Well-aligned carbon nitride nanotubes synthesized in
anodic alumina by electron cyclotron resonance chemical vapor
deposition. Appl. Phys. Lett. 74, 197 (1999).
9. X. Ma and E.G. Wang: CN
x
/carobn nanotube junctions synthe-
sized by microwave chemical vapor deposition. Appl. Phys. Lett.
78, 978 (2000).
10. M. Reyes-Reyes, N. Grobert, R. Kamalakaran, T. Seeger,
D. Goldberg, M. Rühle, Y. Bando, H. Terrones, and M. Terrones:
Efficient encapsulation of gaseous nitrogen inside carbon nano-
tubes with bamboo-like structure using aerosol thermolysis.
Chem. Phys. Lett. 396, 167 (2004).
11. M. Terrones, N. Grobert, J.P. Zhang, H. Terrones, J. Olivares,
W.K. Hsu, J.P. Hare, A.K. Cheetham, H.W. Kroto, and
D.R.M. Walton: Preparation of aligned carbon nanotubes cata-
lysed by laser-etched cobalt thin films. Chem. Phys. Lett. 285, 299
(1998).
12. M. Terrones, P. Redlich, N. Grobert, S. Trasobares, W-K. Hsu,
H. Terrones, Y-Q. Zhu, J.P. Hare, C.L. Reeves, A.K. Cheetham,
M. Rühle, H.W. Kroto, and D.R.M. Walton: Carbon nitride
nanocomposites: Formation of aligned C
x
N
y
nanofibers. Adv.
Mater. 11, 655 (1999).
13. R. Sen, B.C. Satishkumar, A. Govindaraj, K.R. Harikumar,
G. Raina, J-P. Zhang, A.K. Cheetham, and C.N.R. Rao: B–C–N,
C–N, and B–N nanotubes produced by the pyrolysis of precursor
molecules over Co catalysts. Chem. Phys. Lett. 287, 671 (1998).
14. M. Nath, B.C. Satishkumar, A. Govindaraj, C.P. Vinod, and
C.N.R. Rao: Production of bundles of aligned carbon and carbon-
nitrogen nanotubes by the pyrolysis of precursors on silica-
supported iron and cobalt catalysts. Chem. Phys. Lett. 322, 333
(2000).
15. M. Terrones, H. Terrones, N. Grobert, S. Trasobares, W-K. Hsu,
Y-Q. Zhu, J.P. Hare, H.W. Kroto, D.R.M. Walton, P.K. Redlich,
M. Rühle, J.P. Zhang, and A.K. Cheetham: Efficient route to large
arrays of CN
x
nanofibers by pyrolysis of ferrocene/melamine mix-
tures. Appl. Phys. Lett. 75, 3932 (1999).
16. W-Q. Han, P.K. Redlich, T. Seeger, F. Emst, M. Rühle,
N. Grobert, W-K. Hsu, B-H. Chang, Y-Q. Zhu, H.W. Kroto,
D.R.M. Walton, M. Terrones, and H. Terrones: Aligned CN
x
nanotubes by pyrolysis of ferocene/C
60
under NH
3
atomosphere.
Appl. Phys. Lett. 77, 1807 (2000).
17. C.J. Lee, S.C. Lyu, H-W. Kim, J.H. Lee, and K.I. Cho: Syn-
thesis of bamboo-shaped carbon-nitrogen nanotubes using
C
2
H
2
–NH
3
–Fe(CO)
5
system. Chem. Phys. Lett. 359, 115 (2002).
18. R. Sen, B.C. Satishkumar, S. Govindaraj, K.R. Harikumar,
M.K. Renganathan, and C.N.R. Rao: Nitrogen-containing carbon
nanotubes. J. Mater. Chem. 12, 2335 (1997).
19. R. Andrews, D. Jacques, A.M. Rao, F. Derbyshire, D. Qian,
X. Fan, E.C. Dickey, and J. Chen: Continuous production of
aligned carbon nanotubes: A step closer to commercial realization.
J. Chen. Chem. Phys. Lett. 303, 467 (1999).
20. M. Terrones, P.M. Ajayan, F. Banhart, X. Blase, D.L. Carroll,
R. Czerw, B. Foley, N. Grobert, R. Kamalakaran, P. Kohler-
Redlich, M. Rühle, T. Seeger, and H. Terrones: N-doping and
J. Liu et al.: Large-scale synthesis of highly aligned nitrogen doped carbon nanotubes by injection chemical vapor deposition methods
J. Mater. Res., Vol. 20, No. 2, Feb 2005542