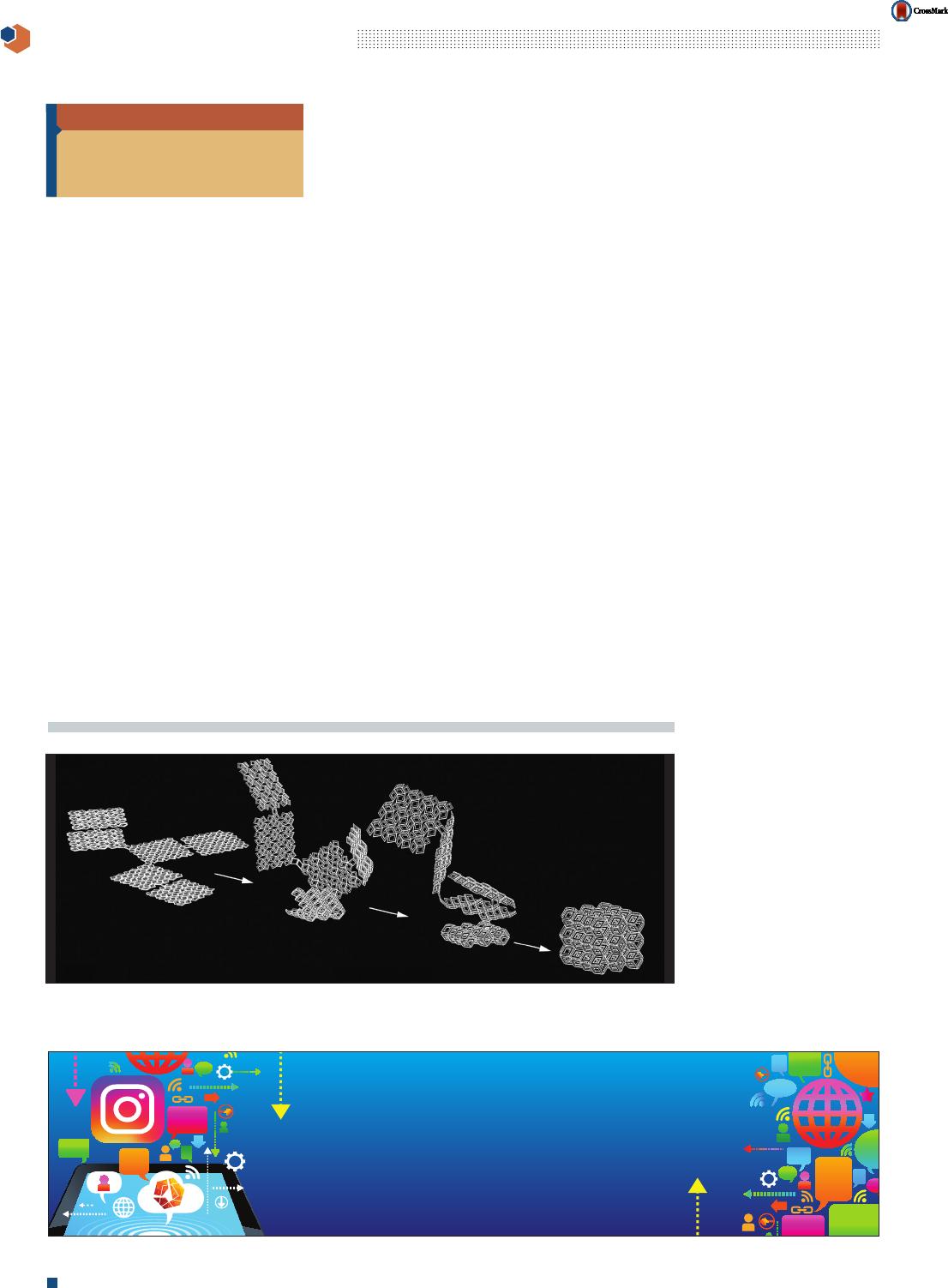
NEWS & ANALYSIS MATERIALS NEWS
166
MRS BULLETIN
•
VOLUME 43
•
MARCH 2018
•
www.mrs.org/bulletin
Origami lattices from flat sheets
and patterning yield metamaterials
with exotic functions
I
nspired by the Japanese paper-fold-
ing art of origami, researchers have
devised techniques to create complex
three-dimensional (3D) lattices from pre-
designed at materials. Unlike 3D print-
ing—one route for creating lattice struc-
tures—the origami method could enable
scientists to add functional features and
create materials with novel properties.
Lattice-like highly porous 3D struc-
tures made of metals, polymers, and
carbon have recently opened up a slew
of novel applications. They can be used
to make ultra-strong, ultralight car and
aircraft parts; for energy storage; as tis-
sue-generating scaffolds in regenerative
medicine; and for metamaterials.
Advanced 3D printing is used to make
lattice-like metamaterials. But it does not
allow easy access to the inner surfaces
of the structures. Accessing the inner
surfaces is critical for creating nanopat-
terns that can stimulate tissue growth or
for integrating electronic sensors inside
metamaterials.
To overcome this problem, biome-
chanical engineers Shahram Janbaz,
Amir Zadpoor, and their colleagues at
the Delft University of Technology in
The Netherlands turned to origami. “We
can start from at structures, use available
patterning technologies to make what you
want on the surface, and then fold it into
the shape you want,” Zadpoor says.
Computational geometry algorithms
allow at shapes to be folded into simple
polyhedra. But there are no algorithms
that create polyhedral lattices, which con-
tain polyhedral cells stacked vertically
and horizontally. The researchers there-
fore devised a general platform for fold-
ing various types of 3D metallic lattices
from at states. They rst identied three
categories of polyhedra, which would be
repeated to make a lattice, and came up
with a folding pattern for each. Then they
gured out how the lattice would need to
be sliced in order to open up completely.
The rst category has the simplest
unit cell—a cubic lattice is a good exam-
ple—and can be sliced along every row
to unfold. The second category has more
complex unit cells, requiring the lattice
to be sliced along the tops and bottoms
of rows. The last category builds on the
second one, by requiring spatial rotations
during the folding process.
As a demonstration, the researchers
hinged together 3D printed aluminum
panels using metal pins and prestretched
rubber bands. The piece self-folds into
a cubic lattice, the team reported in
a recent issue of Science Advances
(doi:10.1126/sciadv.aao1595). To dem-
onstrate the technique’s usefulness for
adding features to the insides of lattices,
they used electron beam deposition to
make tiny 3D structures a few tens of
nanometers in size on the origami sheets,
so that these became situated on the in-
side of the folded lattices. They could
pack multiple types of surface patterns
into the same origami lattice.
“We have demonstrated that we
have a folding strategy,” Zadpoor says.
“Now we want to be able to do this at
the microscale.”
The advantage of this new approach
for creating complex 3D structures is
that it decouples structure
and function, says Jesse
Silverberg, a researcher at
Harvard University’s Wyss
Institute of Biologically
Inspired Engineering. “Most
of the earlier work on origa-
mi-inspired materials shows
that structure is function,” he
says. “Here, functionality
can be arbitrarily prescribed
on the 2D surface before the
structure folds. Once folded,
the functionality is now em-
bedded in the 3D structure.”
Prachi Patel
The self-folding sequences for a rhombic dodecahedron origami lattice. Credit: Science Advances.
Initially at structure
Regular
3D conguration
Join in the conversation and share your
2018 MRS Spring Meeting stories, photos and updates!
Follow #S18MRS on Twitter, Facebook and
NOW on Instagram @materials_mrs.
Phoenix, Arizona
April 2– 6, 2018
|
2018
SPRING MEETING & EXHIBIT
https://www.cambridge.org/core/terms. https://doi.org/10.1557/mrs.2018.45
Downloaded from https://www.cambridge.org/core. IP address: 80.82.77.83, on 26 Apr 2018 at 11:22:58, subject to the Cambridge Core terms of use, available at